Mines researchers provide first dynamic demonstration of kinking mechanism in engineered layered ceramics
Engineered layered ceramics called MAX phases have shown promise in various structural applications in extreme environments, such as cladding in advanced nuclear reactors and high-temperature technologies.
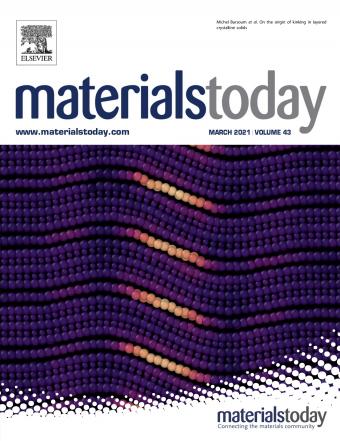
These ceramic materials are stable at high temperatures, more easily machined and less brittle than some other ceramics, and can be produced at lower cost than some refractory metal alloys with similar properties.
But until now, materials scientists and engineers have not fully understood how they respond under pressure.
In findings recently published in the journal Materials Today, researchers from Colorado School of Mines, along with a team of international scientists, provided a multi-scale study of the origins of kinking in MAX phases, linking observed microscale phenomena to mechanisms at the nanoscale. The research team at Mines, composed of Materials Science PhD student and CoorsTek Fellow Gabriel Plummer and Mechanical Engineering Associate Professor Garritt J. Tucker, provided the first dynamic demonstration of the mechanism of kinking in MAX phases using a series of atomistic models and simulations developed at Mines. Their findings confirmed much of the theory that had been around for 70 years while also uncovering some key differences that could help to explain some of the MAX phases’ more interesting properties.
“Kinking is something we see in many natural and engineered layered systems. It could be rock formations or a stack of cards or crystalline materials that are layered at the atomic scale,” said Plummer, the lead author on the paper. “If you take a deck of cards stacked vertically and press down on them, they’ll first bend and then form a kinked structure with sharp boundaries as you press down on them. That’s what we see in a lot of layered materials, when you transition from this very continuous bending structure to all of a sudden getting these sharp-looking boundaries. Kinking is the transition from bending to a sharply deformed structure.”
Classically, scientists have assumed these kink boundaries created an atomically sharp angle in crystalline materials. But through a series of simulations and high-resolution electron microscopy images, the researchers actually observed a pronounced curvature, which only becomes apparent at the atomic scale.
“Instead of breaking or changing their structure, they bend elastically like you would see in a playing card, albeit in a highly localized manner,” Plummer said.
The hope is that scientists and engineers will be able to leverage that fact to engineer the materials’ microstructures to be more ductile in the future.
“If MAX phases did not kink, they would be pretty useless. They’d just crack,” Plummer said. “Understanding it better and understanding the underlying mechanism behind kinking should allow us to better take advantage of that property in designing these materials to make them more suitable for applications.”
The high-level goal is to ultimately engineer these materials to be less brittle so they could potentially replace other materials in high-temperature applications like jet engines and advanced nuclear reactors.
“Once you understand the fundamental mechanisms at play, we can turn the knob on how we engineer these materials for specific applications and future technologies. This new understanding will allow the scientific community to more effectively tune material properties, and structure, by potentially controlling the fundamental mechanisms that ultimately govern those properties,” Tucker said. “That’s the long-term goal, but for the last 70 to 80 years, we’ve relied solely on those pioneering theories that no one has been able to quantifiably support or refute. And now we can.”
Funding for the research came from the U.S. Army Research Office and the CoorsTek Fellowship. Collaborators on the international effort included Texas A&M University, Université Grenoble Alpes (France), Linkoping University (Sweden), nuclear research center SCK CEN (Belgium), University of Huddersfield (UK) and Drexel University.
Read the full paper, “On the origin of kinking in layered crystalline solids,” at https://www.sciencedirect.com/science/article/pii/S1369702120304223#