Meenakshi Singh wins NSF CAREER Award for quantum dot research
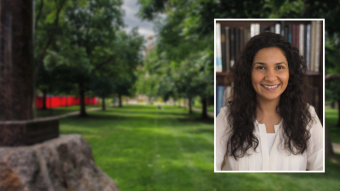
Meenakshi Singh, assistant professor of physics at Colorado School of Mines, has received a National Science Foundation CAREER Award, its most prestigious award for junior faculty, for work on gate-defined quantum dots that could lead to higher operating temperatures for quantum computing devices.
A gate-defined quantum dot is a small region within a semiconductor, defined by applied voltages, where a few electrons can be confined. Acting as “artificial atoms,” these quantum dots are used in widely ranging contexts, from tests of molecule formation to quantum computing.
“For quantum computing, electronic spins confined in quantum dots in silicon are particularly promising because of long coherence times and compatibility with existing MOS processes,” Singh said. “These spin qubits, however, must be operated at ultra-low temperatures, in the scale of milli-Kelvins, to slow down the loss of information due to relaxation and decoherence induced by various effects. This roadblock is at the core of many of the challenges in expanding this technology.”
Singh will receive $685,555 in funding over five years for her project, “Electron-phonon processes in gate-defined silicon quantum dots: measurement, control, and applications.” Singh’s group will directly address the temperature roadblock by studying the ubiquitous coupling between electrons and phonons – mediators for temperature – in quantum dots, and engineering the “ideal” conditions theoretically predicted to lead to “hot” qubits that have good coherence properties at higher operation temperatures.
Here, Singh answers a few questions about her research and how temperature plays an important role in all of her work.
Q: What is your latest research focused on?
A: Electronic spins carry both classical and quantum information. This information is used in computing applications (e.g. spintronics and spin qubits) and preventing its loss is a persistent challenge across fields. One pathway for the loss of the information contained in electronic spins is via interaction with phonons (temperature dependent lattice vibrations) in the substrate where the electrons live. In this project, we will develop methods to control electron-phonon interactions to limit this information loss.
A single or few electronic spins will be confined in a gate-defined quantum dot with the help of applied voltages. Nano-structuring will be used to create a thermally ‘silent’ zone, missing a large number of phononic states, at the location of the quantum dot. The time taken to lose information via relaxation (spin flips) and decoherence (loss of phase information of the electronic state) will be measured at these silent spots. In addition, at specific electron-phonon couplings, spin states particularly robust against decoherence are expected to emerge. In a separate part of the project, these theoretically predicted, decoherence-free subspaces will be investigated by continuous tuning of electron-phonon coupling using strain. Spins with long relaxation and decoherence times, decoupled from the phonon bath, could enable ‘hot’ qubits that have higher operation temperatures than the milliKelvin temperatures typically used for quantum dot qubits today.
Q: What do you find most exciting about your research?
A: As a low-temperature physicist, manipulating temperature is something I think about all the time. My work in both superconductivity and quantum information has further enhanced this interest as low temperatures are (typically) key to the functioning of both systems. So, the possibility of manipulating temperature by engineering local, thermally silent ‘cold spots’ using this novel method is very exciting for me. In addition, this research direction is a convergence of the fields of nanophononics and quantum information, which have not seen a lot of information exchange so far. I believe this fusion of fields will yield surprising insights and new discoveries to explore over the years.
Q: What is the potential impact of this work?
A: Few-spin systems are the fundamental prototype for rationalizing spin dynamics in more complex systems (such as magnetic materials), are important for quantum information applications, and are a fundamental testbed for theoretical predictions of quantum mechanical effects. Understanding and limiting information loss in a single spin (or few-spin) system will therefore have ramifications across many fields. For example, long relaxation times, corresponding to spins that retain classical information for a long time, are important for spintronic applications. Long quantum coherence times, resulting from protection from phonon mediated decoherence (of multi-spin states), are game-changing for quantum computing applications. From a more fundamental Physics perspective, understanding electron phonon thermalization at the few-spin level will be foundational for future quantum thermodynamics studies on the quantum dot platform.
Q: How does this research agenda inform your teaching?
A: In terms of training students in the research lab, both undergraduates (via Senior Design projects and Mines Undergraduate Research Fellowships) and graduate students, this research will provide skills relevant to the nationwide calls for training a quantum workforce including nanofabrication, cryogenics, and microwave measurements. Over the course of this 5-year project, I expect to have 10 undergraduate students and 1 to 2 graduate students work on this research.
In terms of classroom teaching, both the classroom-based courses I currently teach, Digital Electronics and Interdisciplinary Microelectronics Processing Lab, have opportunities for incorporation of what I learn in these research projects into the classroom. For example, the students in the Microelectronics Processing Lab fabricate MOSFETs, which are a giant version of the gate-defined quantum dots I will make for this project. Comparing transport measurements from the MOSFETs operating at room temperature that the students make in the class and the tiny quantum dots operating at cryogenic temperatures will provide an interesting segue into quantum effects. Finally, I participate in Mines’ outreach to middle school children in the Rocky Mountain Camp for Dyslexic Children and will develop modules on electrons, phonons, spins and qubits for that effort.